When you were a kid, what did you want to be when you grew up? An astronaut? An Olympic ice skater? A race car driver? Think back to a time when the possibilities seemed limitless. As you got older, though, you were faced with a cascade of either/or choices: this school, that major, this city, that job. And with each decision, your options became more constrained.
But picture being able to exist in a permanent state of possibility. That’s what makes stem cells so special.
Broadly speaking, stem cells are defined by their capacity for continual self-renewal and their ability to transform into more specialized cells. Almost from the first inkling of their existence, they have been touted as a panacea. Imagine: Tissues or organs that become damaged or dysfunctional due to disease or trauma could be replaced by duplicates grown in a lab from a person’s own cells.
This vision of “regenerative” medicine has yet to be fully realized. Directing these primordial cells to become the specific type of cell that’s needed—and only that type—has proved challenging. And unspecialized cells can have unpredictable effects in the human body. After a quarter century of research, bone marrow transplantation remains the only Food and Drug Administration-approved therapeutic application of stem cells in the United States.
Recently, though, a sense of momentum has been building. Hard-won insights, gained through close study of stem cells’ basic biology and work in animal models, are yielding novel approaches to treating a wide range of maladies. Chief among these are degenerative conditions that affect a specific population of cells in the body, such as arthritis, diabetes, heart disease and neurodegenerative disorders. An encouraging number of new stem cell-based treatments for these and other ailments are progressing through clinical trials. Funding agencies are prioritizing support for this translational, “bench to bedside” research. And changes to the regulatory landscape may mean a shorter time to market for cell- and tissue-based products. With a recently opened laboratory in Palo Alto and plans to support more clinical applications, Stanford is poised to make the most of these developments.
Stem Cell ABCs
There are three main types of stem cells: embryonic stem cells, tissue-specific (also called somatic or adult stem cells) and induced pluripotent stem cells. See illustration, below.
Embryonic stem cells form around five days after an egg is fertilized. At that point, the ball of about 100 cells, called a blastocyst, is no larger than the period at the end of this sentence. These undifferentiated cells were first extracted from mouse blastocysts in 1981 and from human blastocysts in 1998. Embryonic stem cells can replicate indefinitely and, in humans, have the potential to become any of the more than 200 distinct cell types that make up the human body.
Somatic stem cells are the handful of cells in the fully developed body that retain the ability to reproduce themselves and can give rise to a limited number of other tissue-specific cell types. They are the body’s jacks—or jills—of all trades: flexible cells that pitch in as needed to maintain and repair the tissue in which they reside. Hematopoietic, or blood-forming, cells found in bone marrow were the first to be identified, some 50 years ago. But small pools of somatic stem cells have since been found in many organs and tissues of the body, including the brain, skin, skeletal muscle, heart, gut, liver and gonads.
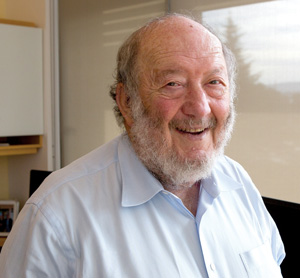
However, for both somatic and embryonic stem cells, getting at the cells can be problematic. With the former, the hurdle is primarily technical. Tissue-specific stem cells are scarce (1 in 100 to 1 in 1,000,000 cells) and interspersed among all the other cell types in an organ. Irving Weissman, MD ’65, was the first person to separate out stem cells of any kind from adult tissue. A professor of pathology in the School of Medicine and former chair of the immunology program, he identified antibodies that would selectively bind to blood-forming stem cells in bone marrow—first in mice in 1988 and then in humans three years later. Members of his lab have since discovered and isolated several other tissue-specific stem cell populations, including a class of brain-forming stem cells in humans and skeletal muscle stem cells in mice.
With embryonic stem cells, the biggest impediments are cultural and political. Nearly all embryonic stem cell lines are derived from extra zygotes created via in vitro fertilization for assisted reproduction. Although most unused zygotes are ultimately destroyed, opposition to such research is motivated by the conviction, often rooted in religious doctrine, that life begins with the fertilization of an egg by a sperm. By those lights, destroying a blastocyst to obtain the cells is tantamount to murder.
In 2001, President George W. Bush cut off federal funding for research involving the creation of new stem cell lines. In response, voters in California and several other states approved ballot measures to direct billions of dollars to support stem cell research. Stanford has been a major beneficiary of the California Institute for Regenerative Medicine, receiving more than 10 percent of dollars granted since its inception in 2004. President Barack Obama in 2009 lifted the Bush-era restrictions, allowing researchers to obtain federal grants for work using cell lines that had been developed in the interim with state or private money. However, a ban on using federal funds to create new embryonic stem cell lines remains in place.
So when scientists in Japan figured out a decade ago that they could take readily accessible cells—blood or skin or fat—from an adult and turn on specific genes that would cause the cells to revert to an undifferentiated state, the discovery was hailed as a neat alternative that sidesteps the issues with both somatic and embryonic stem cells. These genes encode proteins called transcription factors. The factors bind to specific stretches of DNA and tell RNA molecules which sequences to read and which ones to skip. The reprogrammed cells are once again “pluripotent,” meaning that they can be coaxed to become any other type of cell.
But induced pluripotent stem cells have not supplanted embryonic and somatic stem cells in research. With each type having a different set of advantages and drawbacks, the three are complementary.
‘A Huge Surprise’
In 2002, Stanford established the Institute for Stem Cell Biology and Regenerative Medicine with Weissman as its founding director. At the time, says neurosurgeon Gary Steinberg, bone marrow transplantation—where blood-forming stem cells in the marrow literally rebuild the immune system—was the template for how to do regenerative medicine.
“That’s cell replacement,” the founder and co-director of the Stanford Stroke Center says. “And that’s what we thought we were doing with the brain when we were treating stroke or other neurologic disorders” using pluripotent cells. When he first injected neural stem cells from human fetal tissue into the damaged brains of rats 15 years ago, Steinberg, PhD ’79, MD ’80, expected that the cells would turn into neurons and reform circuits. Not only did the introduced cells not integrate, he says, but they also didn’t survive more than a month.
Still, the rats showed clear improvement. The mere presence of the stem cells made the adult brains behave more like neonatal brains—which, Steinberg says, can recover well from injuries, a quality scientists call plasticity. It is now understood that the cells accomplish this by secreting molecules that enhance native mechanisms of recovery, such as axon growth, dendrite branching and blood vessel formation.
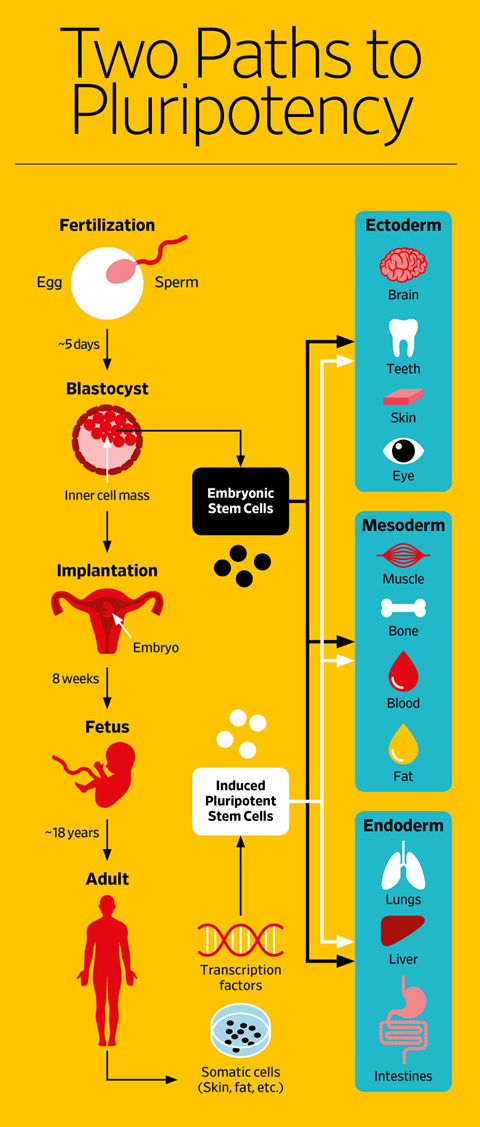
Other researchers have observed similar results when heart stem cells are injected into the coronary artery to treat cardiac damage. “So this is a different idea,” Steinberg says. And it raises the question of whether the type of stem cell used has to match the target tissue.
Last year, Steinberg published the results from a small trial in which he injected bone marrow-derived mesenchymal stem cells—which can form bone, cartilage, muscle or fat, but not brain-type cells—into the brains of people who had suffered a stroke. Typically, he explains, stroke patients improve to the extent that they ever will within six months. After that point, clinical intervention, whether it’s more rehab or a different drug, is unlikely to have any effect. Which means that there are more than 6.5 million stroke survivors in the United States living with significant impairments in mobility, speech and cognition.
The 18 participants in the trial were all well past the six-month mark, with one person five years out; the mean was just under two years. There was no control group. In a sense, Steinberg notes, each patient served as his or her own control, since the chance of spontaneous improvement so long after a stroke is minuscule.
A third of the patients had clinically meaningful gains of 10 or more points compared with the baseline on a 100-point motor function scale. One, a 33-year-old woman, previously had difficulty communicating and didn’t want to get married because she feared she’d be embarrassed walking down the aisle. After the treatment, her speech and mobility improved dramatically; she got married, and she just had a baby, Steinberg relates.
“This was a huge surprise,” he says, and it changes the notion of what happens after a stroke. “We thought the circuits were dead or irreversibly injured, but they’re clearly not; they can be resurrected. We’re still trying to figure out exactly how we are resurrecting them.” As of the end of 2016, Steinberg had already treated five patients as part of a larger multisite follow-up study. This study is randomized and double-blind with controls.
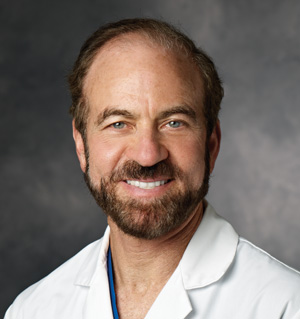
Stem cell treatments for pathologies of the eye, including macular degeneration, glaucoma and corneal damage, also are progressing through clinical trials, says ophthalmology professor Jeffrey Goldberg. “Ophthalmology is one of the fields where we’re out ahead of the curve,” he says, in part because “the eye’s an easy place to get to in patients.”
Macular degeneration and glaucoma are the two main causes of vision loss in the United States, together affecting 4 million people over age 50. In macular degeneration, light-sensing cells in the central part of the retina and the cells that normally help sustain them (retinal pigment epithelium, or RPE, cells) are destroyed. This manifests as a blurry splotch in the middle of an affected person’s field of vision that gradually grows larger as the disease progresses. In glaucoma, increased pressure in the eye causes damage to cells in the retina, called retinal ganglion cells, which collect and pass along information from the light-sensing cells. It also damages the fibers that carry visual information from the eye to the brain. This manifests as a gradual loss of peripheral vision, which can progress to total blindness.
“In both of these we’ve begun to make really significant progress as a field in applying stem cell biology to develop new therapies,” says Goldberg, MD/PhD ’03. Stanford is collaborating as a site of a clinical trial to test stem cell-derived RPE cells in macular degeneration; a therapy to replace the light-sensing cells is still in the preclinical phase.
“One of the interesting elements that we’re learning from the preclinical research and the early clinical trials is that when you just inject stem cell-derived retinal cells into the eye, they don’t integrate optimally into the retina,” he says. That’s likely because the retina is such a complex and highly ordered structure. The introduced cells have to be the right type, and they must also be aligned properly to restore full function.
To address this issue, Daniel Palanker, also a professor of ophthalmology, is working on the transplantation of developed retinal neurons. But glaucoma is an even tougher problem, Goldberg explains, because not only
do you have to replace the retinal ganglion cells, but you also have to get them to grow their axons down the optic nerve and connect to the proper targets in the brain.
Impatient Patients
For their part, patients desperate for relief from debilitating conditions may feel that they can’t wait for the science to be fleshed out. Some are turning to private, for-profit clinics to obtain “off-label” adult stem cell treatments. “I get a call or email every week,” Gary Steinberg says, from someone asking, “Should I go to Russia or India and get some kind of stem cell treatment and pay anywhere from $20,000 to $100,000?” That has many in the mainstream stem cell research community concerned.
There are currently more than 500 of these clinics operating in the United States alone. These businesses advertise stem cell infusions for a wide range of indications. On the cosmetic end of the continuum, there’s antiaging, sexual enhancement and hair loss treatment. But many of the clinics are purporting to treat serious illnesses and debilitating conditions such as chronic pain, pulmonary fibrosis, multiple sclerosis, muscular dystrophy and Alzheimer’s disease.
The majority of the clinics claim to be giving patients mesenchymal stem cells (MSCs, the kind used by Steinberg to treat stroke), isolated from the patients’ own fat, or from their blood or bone marrow. But there’s really no way of knowing what kinds of cells these people are getting, Steinberg says, or if they’re getting any stem cells at all.
People seeking these treatments might assume that the risk is minimal, since the cells come from their own bodies. But if the medical staff at these clinics don’t follow the proper protocols, serious infections, immune reactions or tumors could result. A few reports of bizarre outcomes have surfaced. For example, a Los Angeles woman developed bone fragments in her eyelids after having fat-derived MSCs injected into the area around her eyes as part of a so-called stem cell facelift.
What worries mainstream stem cell researchers is that a major disaster at one or more of these clinics could negatively affect the whole field. They’re putting pressure on the FDA to clarify the criteria governing which cell- and tissue-based treatments are subject to the agency’s oversight. The FDA requires all embryonic or induced pluripotent stem cell products to go through phase I through III clinical trials to establish both safety and effectiveness before they can be marketed to the public. But adult stem cells are a bit of a gray area.
Clinic operators counter that they are technology disruptors, driving innovation. They maintain that their critics in academia and the biotech industry are just out to protect their own financial interests by stifling competition. And patient activists say that denying them access to these treatments is a violation of their human rights.
In September, the FDA held a rare two-day public hearing to gather feedback from stakeholders—academic scientists, clinicians, bioethicists, business owners and patients—on proposed changes to its guidelines covering cell- and tissue-derived therapies. The current rules say that such products are subject to FDA oversight and premarket approval only if they are more than “minimally manipulated”—that is, if they undergo processing that fundamentally alters the “relevant biological characteristics of cells or tissues.” The new language being considered explicitly points to MSCs isolated from fat as an example of more than minimal manipulation. The FDA has not given a timetable for updating the guidelines.
Meanwhile, the 21st Century Cures Act was passed by the Senate and signed into law by President Obama in December. Among other things, it lets companies apply for a new “regenerative advanced therapy”designation for stem cell and gene therapy products. This designation makes them eligible for several existing types of special consideration. They can, for example, be granted “accelerated approval” based on evidence other than “demonstrated clinical benefit,” such as reduced symptoms. Additional studies to prove that the treatment works would still be required, but they would be done after the product was already on the market.
Pushing Ahead
Maria Grazia Roncarolo, professor of pediatrics and medicine, praises the move. “Any political step that can be taken to accelerate the use of these approaches to save lives and really give a therapeutic opportunity to patients with a high unmet medical need is extremely important,” says Roncarolo, who’s also co-director of the Institute for Stem Cell Biology and Regenerative Medicine.
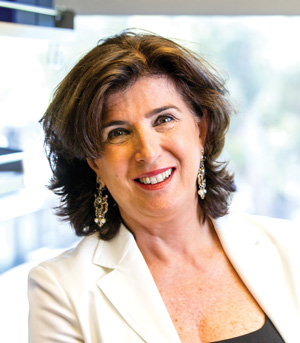
While serving as scientific director of the San Raffaele Telethon Institute for Gene Therapy in Milan, Italy, Roncarolo successfully brought to fruition a number of products using stem cells to treat patients with genetic diseases. The first, licensed to GlaxoSmithKline, received European authorization for commercialization in 2016.
When she was considering the move to Stanford, Roncarolo visited the campus and says she was blown away by “the advanced technology, cutting-edge science and pipeline of potential products to be translated.” She noted, however, that what was lacking—not just at Stanford, but at academic institutions in general—was “a systematic effort” to translate the basic science into novel treatments.
“No single person has done as much as she in this field, or as successfully,” Weissman said in 2014, when announcing her appointment.
“That is the reason why I was recruited,” Roncarolo says. “My mission is to help the university to build a brand in stem cell therapy, not only from the fundamental discovery but also for the clinical application.”
Speaking in early January from her office in the Lorry I. Lokey Stem Cell Research Building, Roncarolo explains that it’s a busy time for the institute, which she co-directs with Professor Michael Longaker. “We are in the middle of building not only a strategic plan but also the infrastructure to catalyze the resources to really help investigators to accelerate this translation.”
One essential milestone in building that infrastructure was realized last fall when a building on California Avenue in Palo Alto reopened its doors as the Stanford Laboratory for Cell and Gene Medicine. The LCGM is Stanford’s first dedicated facility equipped to produce cell-based therapies in compliance with the FDA’s stringent “good manufacturing practices” for use in human patients.
Other plans include establishing an office dedicated to helping investigators prepare investigational new drug filings to get FDA approval for human stem cell trials, and a dedicated clinical unit for pediatric patients where investigational trials will be conducted.
“What we are now pushing into the clinic is just the tip of the iceberg,” Roncarolo says. “But what we really want to build is a translational research program that would allow all the potential stem cell therapies to surface and be offered to patients in need.”
Versatile Lab Helpers
“The regenerative medicine aspect, in my opinion, is more difficult and that’s a longer-term goal,” says Joseph Wu, a professor of cardiovascular medicine and director of the Stanford Cardiovascular Institute. But there are other ways that stem cells—specifically, induced pluripotent stem cells—can improve health outcomes for patients right now.
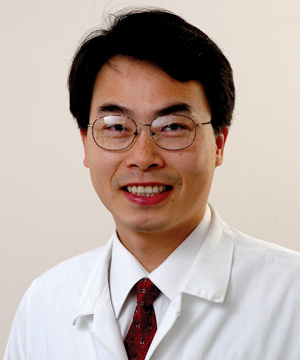
Disease Modeling
Wu has generated iPS cells from patients and differentiated them into heart cells to study the pathology of several common types of heart muscle disease as well as syndromes that cause sudden cardiac death. Understanding the mechanism of a disease is a prerequisite for identifying potential therapeutic targets, he says. This approach could replace animal models, or at least be another option in cases where there isn’t a model for a disease.
Precision Medicine
A well-documented side effect of the chemotherapy drug doxorubicin is that it causes cardiac damage in about 10 percent of breast cancer patients. In the past, doctors have had no way to predict whether a given patient will develop heart failure. Wu is using iPS cell-derived heart muscle cells that express individual patients’ genetic makeup and disease phenotype as “an in vitro diagnostic kit to figure out who’s going to develop toxicity and who’s not.”
Drug Development
The FDA requires pharmaceutical companies to test all drugs for cardiac safety. One flaw with the current method, Wu says, “is that the screening is so sensitive that it’s killing a lot of potentially good drugs.” He envisions creating a biobank of iPS cell-derived heart, brain, liver and kidney cells from a diverse cross-section of people to test and compare the safety profiles of various drugs—essentially, a “clinical trial in a dish."
Greta Lorge, ’97, is a writer and an editor in the Bay Area.