Jeanne Paz sat in the dim light of the basement laboratory, staring intensely at her research subject. The rat took little notice, and continued nosing about the bedding of his cage. Paz checked the EEG monitor, watching the waves of electrical activity coming from the rodent's brain. Suddenly, the once wobbly waveform began to spike rapidly, becoming a dense forest of zigzag lines.
Even to an untrained eye it would have been obvious that the rat's brain was acting very strangely. To Paz, it was a clear sign of an epileptic attack. Indeed, the rat was no longer casually exploring his enclosure, but was struck motionless, frozen in a corner of his cage. After nearly a minute, the wild spiking of the EEG signal tapered off, and the rat relaxed, returning to his normal rodent business.
A few minutes later, the EEG signal began to spike again with the onset of another attack. This time, however, Paz flipped a nearby switch, and the epilepsy-like activity resolved instantly. The rat seemed unaware that anything out of the ordinary was happening, but Paz felt the hairs on the back of her neck stand on end. "In French we call this chair de poule," Paz told me later. "Goose pimples."
She raced to the phone to call her adviser, neurology professor John Huguenard, who was away at a conference. "I was shaking," Paz remembers. "I thought I would faint." When Huguenard answered, she exclaimed, "Oh my God, I was able to stop the seizures! It's amazing!"
"I remember the excitement in Jeanne's voice, certainly," Huguenard recalls. "I think she was more or less saying, 'See John, I was right.'"
Seizures occur when the normal pattern of electrical signaling between neurons goes haywire and then spreads across the brain, like a single spark catching a dry forest ablaze. Much effort in epilepsy research has gone into studying where seizures begin, in the hope that neurologists can put out that spark—using drugs, implanted electrodes or even surgical excision—before it spreads.
Paz was several years into her postdoctoral research at Stanford, studying a rat model of a type of epilepsy that often develops following stroke, when she made an unexpected discovery that could change how neurologists think about how to treat the disease. She noticed that neurons in a brain structure called the thalamus, which acts as the brain's central router of information, started behaving erratically a few weeks after the rats suffered a stroke, despite being located some distance away from where the seizures presumably originated.
This gave Paz an unusual idea. What does it matter where seizures start, she asked herself, if our objective is to stop them? Instead of trying to stamp out the spark of aberrant activity that leads to seizures, often at the expense of healthy brain tissue, why not focus on stopping seizure activity from spreading to the rest of the brain?
Coming from a family of physicists—including her parents, uncles, aunts, cousins and cousins' spouses—everyone thought Paz would follow suit. But early brushes with the violence of war convinced her to take a different path. At age 10 Paz was living in Israel, where her parents worked at the University of Tel Aviv, when the Gulf War started. She remembers carrying a gas mask every day on her walk to school and hiding with her family and neighbors in bomb shelters most nights. Then, at age 12, she went to live with her grandmother in Tbilisi, Georgia, just as civil war broke out. Paz saw her young cousin hit by a stray bullet. "He spent years in the hospital, and I wished I was a doctor to understand how to make him feel better."
The cousin recovered and went on to become a physicist like the rest of the family. Paz, meanwhile, graduated from high school early and enrolled in medical school in Lyon, France. Once there, however, she found herself frustrated by the emphasis her professors placed on rote learning. "They said it would be better for me to do science," she says with a laugh. "I think they were probably annoyed by the thousands of questions I was asking." So, at the age of 19 she left her medical training and moved to Paris to pursue a doctorate in neuroscience.
SENSE OF THE POSSIBLE: Huguenard calls Paz 'fearless.' (Photo: Linda A. Cicero)In graduate school, Paz developed a passion for the study of epilepsy, which gave her a way to help people suffering from a debilitating disease as well as a window into understanding normal brain function. Unlike many neurological diseases, where damage and dysfunction occur gradually and can be hard to tease apart, "the epileptic brain can be absolutely normal between seizures," she says. "So what really fascinates me is how the brain goes from a normal state to an abnormal state. What is really required for the normal functioning of the brain?"
After earning her PhD studying the behavior of cells in living animals with epilepsy, Paz wanted to learn more about the mechanisms that cause the disease. She applied to become a postdoctoral researcher in the Huguenard lab at Stanford, known for its careful explorations, using slices of brain tissue, of the cellular interactions underlying seizure initiation and spread.
John Huguenard's office is a small, windowless room centrally located among his students' desks and physiology rigs, from which tumble birds' nests of electrical wires and latex tubing. His open door policy means that any of his students or postdocs can drop in anytime to talk about research ideas or problems. It also means that he can venture out frequently to troubleshoot a rig or look at the latest results on a student's screen. The former director of Stanford's neuroscience graduate program (he stepped down at the end of 2013), Huguenard is also known for his rotation of outlandish whiskers, his inscrutably dry sense of humor and his penchant for commuting to work by Rollerblade.
He has spent much of his professional career in this basement corridor, where he still shares laboratory space and sometimes students with his former postdoctoral mentor, David Prince. Students may complain about the lack of sunlight, but deep in the bowels of the Medical School is one of the best places to obtain stable recordings of the subtle electrical language of neurons.
Nearly all the sensory data your brain takes in—the sound of a bird chattering outside your window, the sight of the sun setting behind the hills or a friend's gentle touch on your arm—passes through a structure called the thalamus on its way to the cortex, the outermost wrinkled layer of brain tissue that most neuroscientists consider to be the seat of perception and higher cognition. Huguenard thinks the thalamus acts as a sort of amplifier, allowing the cortex to adjust the volume of the incoming information and perhaps even to select the most salient details. As with an audio amplifier, however, if the volume is turned up too high or the wiring is faulty, the whole system can squeal with a vicious cycle of out-of-control feedback.
Huguenard and others in the field have long wondered what changes in the brain cause the normally harmonious communication between these two regions to fall into discord. When Paz first arrived at Stanford, Huguenard asked her to investigate how brain damage caused by a stroke can lead to epilepsy weeks or months later. To do this, she first had to learn how to carefully and consistently cause targeted strokes in the cortices of a group of lab rats. Then, later, she would make brain slices to look for changes in the thalamic neurons' behavior.
The first thing she observed was that over the course of several weeks, the damage caused by the stroke seemed to spread from the cortex to the thalamus. Some of the cells in the structure started behaving oddly, firing in response to much less input than usual and producing bursts of activity that looked almost like a seizure. It wasn't just the damaged cortex failing to use its thalamic amplifier properly; in brain slices these bursting thalamic neurons were completely cut off from their connections to the cortex. "I knew something was weird in the circuit," she says.
In this case, weird was good. It meant she was on to something. Paz wondered if the transformation of these thalamic neurons might play a role in the development of post-stroke seizures—amplifying and spreading faulty activity from the damaged brain region to other parts of the brain. If so, she predicted she might be able to block the spread of the seizure in a living animal by temporarily deactivating this small but highly influential structure.
The thalamus acts as a sort of amplifier. But if the volume is turned up too high or the wiring faulty, the system can squeal with out-of-control feedback.
To test her theory, Paz proposed an ambitious experiment. First, she would induce cortical strokes in some rats. Then, using optogenetic techniques developed by Stanford bioengineering professor Karl Deisseroth, PhD '98, MD '00, she would genetically modify neurons in their thalami to go silent when exposed to yellow light. Next, she would implant devices to let her shine a laser beam into this deep brain structure. Then she would wait, monitoring cortical activity with EEG electrodes, for the four months it typically takes for one rat in three to develop seizures after stroke.
As her adviser, Huguenard was dubious. He worried that it wouldn't be possible to precisely target the exact place in the thalamus that was affected by the cortical stroke. And even if this technical obstacle could be overcome, he suspected that the seizures might be too widespread to block by targeting a single location. There was no reason the seizure couldn't spread through the cortex itself, for example. "[Paz's] hypothesis—that you could just take one small part of the brain and shut it down and block the seizures—seemed like a stretch," he recalls. "I considered it a remote possibility."
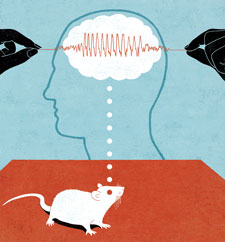
"Saying no is not his style," Paz says of Huguenard. He merely advised her not to be too disappointed if nothing came of her attempt. Then he put all of his support behind the plan, helping Paz connect with collaborators Tom Davidson, a postdoc in Deisseroth's lab, and neurologist Eric Frechette. The team set about designing a device that would use real-time EEG to automatically detect the onset of seizures and activate the optogenetic thalamic blockade. "It was there that it was clear how Jeanne was evolving [as an independent researcher]," says Huguenard. "She was taking the lead in these discussions about how the project was going forward, with me taking less and less of a role."
Months went by before Huguenard got the phone call from an ecstatic Paz, who had managed to halt a seizure in an epileptic rat on her very first try. She subsequently showed that she could reproduce the effect dozens of times in two different rodent subjects. Despite the small sample size, the experiment vividly demonstrated the thalamus's involvement in propagating seizures in awake, behaving animals. A paper detailing her findings was accepted by the prestigious journal Nature Neuroscience and published in May 2013.
Huguenard is eager to explore the exciting new questions this research raises. The fact that blocking thalamic activity works so completely against seizures that probably originate in the stroke-damaged cortex suggests that this could ultimately lead to a whole new array of treatments for epilepsy in human patients. Since the manipulation only briefly targets the thalamus, "any cognitive processes that are occurring in cortical circuitry will be completely unaffected," he says.
"Nonspecific treatmentswith lots of side effects are a big problem clinically," agrees Paul Buckmaster, a professor of comparative medicine at Stanford who also studies epilepsy but was not involved in this project. He says Paz and Huguenard's discovery is especially groundbreaking because it demonstrates "a treatment that is more selective than anything currently available for patients."
Nevertheless, both Paz and Huguenard stress that the particular optogenetic approach Paz used—fundamentally modifying neurons at the genetic level to make them sensitive to light—is probably not suitable for use in humans anytime soon. Other techniques, however, such as deep brain stimulation, which is already approved by the FDA for use in Parkinson's disease patients, could possibly be employed to inhibit the thalamus of epilepsy patients, Huguenard speculates.
Since her paper was published, Paz has been interviewing for research faculty positions around the country while still juggling at least 10 projects in the lab. She plans to continue applying the basic idea she developed during this study to different forms of epilepsy.
"She's going to do amazing things," says Huguenard. "Science is a combination of luck, hard work, creativity and good taste," he explains. Paz has all of these traits in spades, but Huguenard can point to one quality that sets Paz apart: "Fearlessness," he says. "She started fearless and has become even more fearless. She has a sense of what is possible rather than what is going to be difficult."
For her part, Paz hopes that she can be as supportive and inspiring a mentor to her future students as Huguenard has been for her. "You have to become a psychologist," she laughs. "You have to know who you're dealing with and how to interact with them to make them successful." Looking back, she says of Huguenard, "he probably thought a lot of my ideas were crazy, but he trusted me."
Nicholas Weiler, PhD '14, is a Christine Mirzayan Science & Technology Policy fellow at the National Academy of Sciences.