It is tempting to cast cancer, a disease that claims more than 7.6 million lives worldwide each year and impacts many millions more, as a villain. But, as physician and author Siddhartha Mukherjee, '93, notes in his Pulitzer Prize-winning "biography," The Emperor of All Maladies, we'd do well to acknowledge that "cancer is a phenomenally successful invader and colonizer in part because it exploits the very features that make us successful as a species or as an organism." Chief among these is the ability to adapt to inhospitable environments. Aggressive, rapidly-dividing tumors outgrow their blood supply and become starved of oxygen. But where normal cells wither and die in low-oxygen (hypoxic) conditions, cancer cells display extraordinary inventiveness in the face of obstacles to their survival.
"Hypoxia is a driving force in terms of solid tumor development and progression," says Amato Giaccia, a professor of radiation oncology at the School of Medicine who has spent the past two decades studying how tumor milieu drives cancer cells' evolution. Hypoxic cells undergo a myriad of molecular changes that not only allow them to survive, but also make them more aggressive, more prone to metastasize and more difficult to treat via conventional methods.
Yet these changes present "a potential Achilles' heel of cancer cells," says Giovanni Melillo, an expert in tumor hypoxia who was at the National Cancer Institute for 20 years before joining Bristol-Myers Squibb in 2011. Because normal tissue is not hypoxic, drugs that attack hypoxic cells exclusively should have few systemic side effects. Since the 1960s, a number of research groups have tried to develop drugs that exploit hypoxia. "Stanford is definitely one of the leading schools in this field. And Amato's group, without reservation, represents one of the leading groups," Melillo says.
While others have targeted specific adaptations in the hypoxic cell, with limited success, Giaccia's group has taken a different line of attack. Giaccia studies renal cell carcinoma, the most common form of kidney cancer. Most renal tumors have a telltale genetic mutation—loss of the tumor suppressor gene VHL—that makes the cells think they are hypoxic and turns on the same suite of biological changes. Using this model, Giaccia's lab has developed several promising cancer drugs that strike a diverse array of hypoxia-related targets.
Though still in preclinical or early clinical testing, the drugs offer hope for particularly deadly cancers, including renal, pancreatic and ovarian. What's more, Giaccia is innovating ways to drive the development of these drugs from the lab to patients.
ONCOLOGY ODYSSEY: Giaccia is on a quest to develop therapies that exploit cancer's Achilles' heel. (Photo: Duncan Stewart / School of Medicine)Without oxygen, cells can't produce enough energy to live. Mitochondria, the cells' power plants, use oxygen to convert sugar into energy—a process called aerobic metabolism. But hypoxic cancer cells cleverly switch to an alternate energy-generating process that doesn't require oxygen. Called glycolysis, this process is 100 times faster than aerobic metabolism, so it provides quick fuel for rapidly growing tumors. But it is inefficient, requiring nearly 20 times more sugar for the same energy payoff. Thus, excessive sugar consumption is a hallmark of many solid tumors. This is how doctors can see tumors on a PET scan—they attach a radioactive tag to the sugar glucose, inject it into a patient, and look to see which cells gobble it up.
"There's a tremendous promise to kill cells that have become addicted to this process of glycolysis for their energy," says Patrick Sutphin, MD/PhD '07, who was a doctoral student in Giaccia's lab. Researchers have previously tried to exploit cancer's sugar addiction using glucose analogs—molecules that look similar but don't yield energy. However, these drugs turned out to be highly toxic, because normal cells also need glucose to function.
In 2004, Giaccia's team performed a massive hunt for drugs that would specifically kill VHL-deficient cancer cells and leave others unharmed. "It was an exciting time for us," says Sutphin, who spearheaded the effort. The team took advantage of the newly installed High-Throughput Bioscience Center in the basement of the Center for Clinical Sciences research building. "We were actually the first group to do a major screen on their automated robotic system," says Sutphin, now a clinical fellow in radiology at Massachusetts General Hospital. Starting with 64,000 chemical compounds, they found about 200 candidates that specifically destroyed VHL-deficient cells; from there, they whittled it down to the two most potent killers. "When we finally came down to those two drugs that actually worked that was a pretty good moment," Sutphin says.
The drugs were selected based solely on their killing proficiency, without regard to mechanism. However, it turns out that one of the drugs, STF-31, kills precisely by starving hypoxic tumors of glucose. Glycolysis-dependent cancer cells mass-produce a protein that helps shuttle glucose into the cell's interior. STF-31 binds to and disables this protein, turning off the flow of glucose. The drug dramatically slowed tumor growth in mice with renal cell cancer, Giaccia's team reported in Science Translational Medicine in 2011. And since normal cells don't depend on this particular protein for glucose transport, it should be safe and effective against a variety of types of cancer, adds Denise Chan, PhD '04, who was co-first author on the paper, along with Sutphin.
STF-31 offers another key advantage. With most cancer drugs, clinicians may need weeks or months to gauge how well the treatment is working for a particular patient. But with STF-31 they can immediately watch the drug in action using a PET scan. If the tumors suddenly begin guzzling less glucose, this will be visually apparent. "Basically we're able to directly monitor whether or not this compound is functioning," says Chan, now an assistant professor of radiation oncology at UCSF.
Cells have a natural recycling system, a way of breaking down worn-out cellular machinery so the parts can be reused. Cells sequester the defunct materials within a bubble called a vacuole, where they bombard them with digestive enzymes. Hypoxic cancer cells take advantage of this process to fuel growth when resources are low. "It's a coping mechanism for the cell to try to recycle some of its building blocks and survive," Chan explains. The mechanism, called autophagy, can be a double-edged sword, though: Cells can literally eat themselves to death. "If it's prolonged, the cell eventually dies. If you saw a picture, these large vacuoles basically engulf the whole entire cell from the inside out."
Unexpectedly, the second hit from Sutphin and Chan's drug screen, STF-62247, works by triggering cells' recycling regimen. "We would never have guessed that this would have been a mechanism by which we could kill renal cancer cells. But finding new mechanisms was one of the great opportunities of this drug screen," Sutphin says. In mouse studies, the compound stopped renal tumor growth without harming normal tissue.
Whether the drug can be applied to other types of cancer is still uncertain. Because autophagy can have a pro-tumor function, dialing it up might actually benefit some cancers. "It's kind of this Janus-faced process, and it's unclear: Do you really want to disturb it or not?" Giaccia says.
STF-31 and STF-62247 are in preclinical testing with Ruga Corporation, a company that Giaccia co-founded in 2008. The drugs could be ready for clinical testing in as soon as two years, he says.
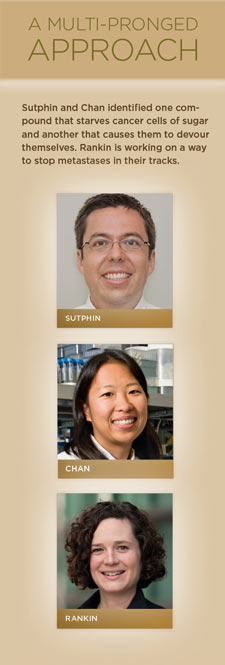
Solid cancers start life as a cohesive mass of cells with smooth edges. In this state, a surgeon can scoop out the tumor and cure the patient. But eventually some cells break ties with their neighbors, crawl along the surrounding connective matrix and burrow into nearby tissues. Ultimately, they infiltrate the blood or lymphatic systems, which carry them to other parts of the body, making the cancer much more difficult to eradicate. "Metastasis is what kills most patients. So if we could prevent tumors from metastasizing to distant sites, that would have the biggest impact on patient survival," says postdoctoral fellow Erinn Rankin.
Hypoxic cancer cells are better escape artists than other cancer cells. For example, hypoxia spurs a cell's inclination to wander, so that it can migrate to more oxygenated areas. In 2003, Giaccia's team began hunting for the genes involved in hypoxia-induced metastasis. A microarray experiment turned up hundreds of genes that change their activity when oxygen is low or when VHL is turned off. From there, they identified two genes with clear roles in metastasis.
In 2006 papers in Nature and Cancer Research, Giaccia's team showed that hypoxic cells overproduce a protein, dubbed LOX, that helps organize connective tissue. When overproduced, LOX creates tracks on which cancerous cells travel—a kind of "highway to metastasis." High levels of the protein predict metastasis and death in breast cancer patients. And inhibiting the protein with an antibody blocked metastasis in a mouse model of breast cancer.
Giaccia co-founded a company, Arresto Biosciences Inc., in 2007 to develop and test this LOX inhibitor. Gilead bought the company in 2011, and the drug is in early clinical trials in pancreatic cancer patients.
"LOX was really the first big target that started all of this," says Rankin, who joined Giaccia's lab in 2007. She immediately became interested in another hypoxia-induced gene: AXL. "We knew that this protein stimulated migration and invasion in normal cells, so we wondered if it might also play a role in cancer metastasis." Sure enough, she found that the protein was highly expressed in a variety of metastatic human cancers, including ovarian and breast.
AXL is a receptor. Embedded in the cell membrane, it acts like a lock. Rankin designed a molecule that looked enough like AXL to fool the protein "key," that normally activates it. The decoy acts like a magnet—attracting AXL's binding partner and keeping the real receptors unoccupied.
In mouse models of ovarian cancer, the drug completely blocked the progression of disease. "That was amazing," Rankin says, "because these animals normally have metastases everywhere in their abdomens." Treated mice experienced no side effects, and mice genetically engineered to lack AXL appeared completely healthy. "So we've been very encouraged," she says, adding that the therapy would likely work in other cancers that express the protein, including renal, breast and lung cancer.
Giaccia's lab recently teamed up with a protein engineering group at Stanford to improve the drug. In collaboration with Jennifer Cochran, assistant professor of bioengineering and chemical engineering, they increased the drug's binding affinity at least 20-fold. "And biologically it appears to be better," Giaccia says.
Cancer is a mini evolutionary system. Disarm one molecular feature, and cancer can evolve another workaround. For example, if one glucose channel is blocked, cancer cells may crank up production of an alternate channel. Thus, despite the promise of targeted therapies, many patients respond initially only to later succumb to their disease. In metastatic renal cancer, newer targeted therapies have incrementally extended patients' lives, but haven't cured them, Giaccia says.
The drugs coming out of Giaccia's lab are particularly promising because they strike very disparate pathways. When given in combination, they may be able to disarm cancer cells beyond their ability to evolve. They also target some key cancer survival tricks that have not been successfully targeted previously, opening new avenues for treatment.
Of course, it's still too early to know if the drugs will work in people. They represent an "excellent, wonderful proof of principle," Melillo says. But drugs that work beautifully in cells and animals often fail in patients, because preclinical models can't capture the complexity of human cancer. "Tumor tissue from the same patients in different locations can have such heterogeneity you would almost think that they are different cancers," he says.
Giaccia worries about a more insidious reason that the drugs may fail: They may languish in the gap between bench science and patient care. "In the past 30 or 40 years, we've made enormous progress in understanding the fundamental biology of cancer cells, but the therapy aspect has really lagged behind," he says. Academic labs develop the blueprints for targeted weapons against cancer, but they aren't equipped to take drugs through clinical trials. Researchers may sell their ideas to outside companies, but this isn't optimal for the science. "When the goal is driven by looking for return on investment rather than increases in efficacy, there can be a much different result."
Giaccia is innovating alternative solutions. "One of the things I'm particularly interested in is thinking about new ways of bringing things to the clinic without giving up control." By retaining control, his team could ensure that the drug was developed in the context of the fundamental biology. For example, they could anticipate ways that the cancer might evolve resistance, and create drugs that target these escape maneuvers.
The small independent companies Giaccia has co-founded to take drugs forward afford him an advisory role, but he envisions more radical approaches such as establishing an entity within the Medical School that would be capable of designing molecules and taking them into the early clinical stages. "Don't be fooled, there's a lot of risk with that. It could fail," Giaccia says. "On the other hand, if we don't try these types of approaches then we're going to be locked into the traditional way."
Kristin Sainani, MS '99, PhD '02, is a clinical assistant professor in the department of health research and policy.