For years, Polly Fordyce has been irked by a chasm separating the worlds she straddles, bioengineering and biology.
On one side, bioengineers—especially in her field of microfluidics—too often develop cutting-edge devices that they demo using yawningly simple biological systems that have been “studied to death.” On the other, the biologists who might put such tools to truly trailblazing use are left to pine; they don’t have the skills to customize the technology themselves, and engineering labs have scant academic incentive to do it for them. Nobody earns a PhD, or tenure, adapting devices for others.
“There are devices that have the potential to really push biology forward, and they often don’t achieve their maximum potential because there is this gap,” says Fordyce, PhD ’07, an assistant professor of genetics and bioengineering. “It kind of frustrates me.”
So when Fordyce readied to teach BioE301D for the first time winter quarter, she wanted to do more than teach grad students how to design and fabricate microfluidic devices, which precisely manipulate fluids at microscopic scales. She wanted to build a bridge.
Fordyce sought out students within and without bioengineering and put out queries to colleagues in the Medical School and the biology department seeking ideas for devices they longed to have.
She got nine replies from scientists, who soon stood in front of her class, vying to persuade one of four teams of students to bite on their proposals. Postdoctoral researcher Cawa Tran was among those scientists. Soon to join the faculty at Cal State-Chico, Tran and her fellow researchers wanted to be able to isolate and image larvae of the sea anemone Aiptasia, a relative of coral.
Their research has obvious real-world applications, including a better understanding of coral bleaching, a devastating consequence of warming oceans. But the squishy, tiny larvae are strong swimmers and not easily wrangled under the microscope. And so samples are usually dead, which limits what can be studied.
As Team Traptasia, which chose Tran’s project, began looking for a microfluidic solution, one of its guiding lights was keeping the design simple, says Will Van Treuren, a third-year PhD student in microbiology and immunology. Operating in dimensions smaller than the diameter of a human hair, microfluidics exists in a nonintuitive world where fluids don’t behave as they would at a macroscopic scale.
Van Treuren had previously tried integrating microfluidics into his research isolating microbes in the human gut. But without expert guidance, he struggled. After Fordyce’s class, he says, he’s prepared to build basic devices himself. Equally important, he now has a shared language to initiate collaboration with bioengineers. “It brings you up to the base level to cross the divide,” he says.
Not that the class is in any way remedial for the engineers involved. Standing in a clean room, in head-to-toe covering, Louai Labanieh, a first-year bioengineering PhD student, points out the 64 microscopic valves within one of Team Traptasia’s designs. If one of the many trapped larvae shows an interesting reaction, it’s possible to use the valves to move it aside for further inspection.
Fordyce sees educational value in working on real-world projects. Doing so requires students to tackle open-ended questions, where success is not assured. Actually solving the clients’ problems wasn’t something she necessarily expected.
If the students had just designed and fabricated devices that worked from an engineering perspective, that would have been a success, she says. Instead, all of the projects made headway on the clients’ wants, perhaps most notably Traptasia, which created a sort of obstacle course that snugly trapped the larvae in open-ended chevrons, securing them for hours and allowing scientists to observe their reactions to different stressors, such as warm water.
“It’s exceeded our expectations, and my wildest dreams, really,” Tran says. “I can honestly say it’s going to be a game changer for the field. I don’t think this opportunity would have come up for me if it weren’t for this class.”
THE PROJECTS
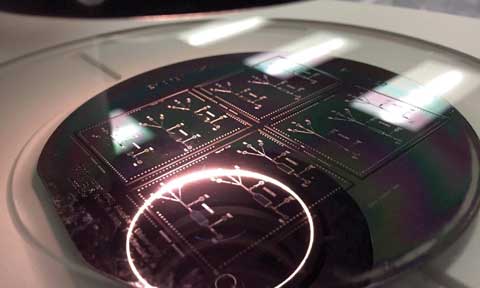
Team 1 (Team Toxo)
This team created a device that grows human cells in a microscopic chamber before exposing them—at different flow rates—to toxoplasma, one of the world’s most common parasites, to see how the pathogen attaches to and invades human cells. Long-term, the project seeks to introduce mutant versions of toxoplasma to examine what genes and which proteins best explain why the parasite is so pathogenic in humans. Above is a 4-inch wafer the team used to cast its microfluidic devices.
Team 2
This team’s aim was to trap and sort placental cells, which are less understood than much of the human body. Their medical school collaborator is particularly interested in big placental cells and would like to do some of the first explorations into why these cells are so important in development and in helping provide the fetus with oxygenated blood and nutrients. The team created a device with thousands of tiny pillars to trap cells from 10 microns (a 10th of the diameter of a human hair) to 150 microns in diameter.
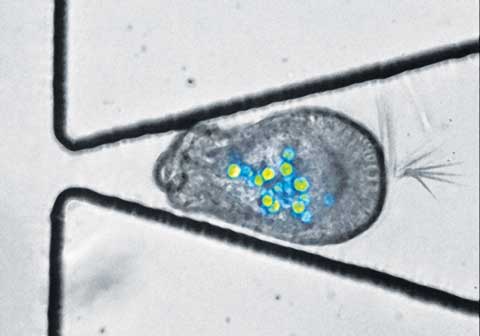
Team 3 (Team Traptasia)
This team successfully trapped the elusive larvae of sea anemone, a cousin of coral, using an array of open-ended chevron-shaped traps (above). Researchers want to observe the larvae’s response to stressors, such as warm water, which can cause it to eject the algae living symbiotically within it. Such ejections are devastating to the coral and are a cause of coral bleaching.
Team 4
This team focused on creating a device that is capable of isolating and performing molecular biology reactions on single cells. Their project involves creating little reaction chambers, where certain types of interesting cells, like cancer or immune cells, can be trapped and where researchers can add in reagents to break those cells open and look at their RNA transcripts to assess how those cells are different from other types of cells.