It can be incredibly frustrating and disheartening, particularly for people who have been personally affected by cancer, to read about yet another “next big thing” only to realize that even a bona fide scientific breakthrough does not guarantee that a blockbuster drug or treatment is in the offing. The all-too-common delays and disappointments are tough on the researchers involved, too.
On April 29, 2010, Edgar Engleman was in Washington, D.C., attending a medical conference. While chatting with a mixed group of academics and industry people between sessions, he heard the news: The first-of-its-kind therapeutic cancer “vaccine” Provenge had been approved by the FDA. It was more than 20 years since its discovery—and cause to celebrate for the company Dendreon’s investors as well as for patients with prostate cancer, many of whom had lobbied loudly for access to the treatment.
For Engleman, an immunologist in whose Stanford lab the pioneering approach had originated, learning that Provenge had finally been approved was one of those moments that become permanently etched in memory. “I remember where I was when Kennedy got shot. I remember where I was in the ’89 earthquake. And that’s about it, those three things,” he says.
Unfortunately, winning FDA approval was not the end of Dendreon’s struggles, and Provenge has not been an unqualified success. For one thing, it’s incredibly expensive to produce because the vaccine has to be customized, using each patient’s own white blood cells.
Engleman has had little to do with the company since its founding, having opted to remain at Stanford to focus on basic research. But he has continued to pursue the idea of activating the immune system to fight cancer. The question now, he says, is whether there is “a way of targeting [immune] cells in the body, and doing better than we were doing manipulating the cells outside the body—not just from a cost perspective but from a potency perspective.”
The answer appears to be yes.
Imagine, if you will, what would happen if you left an unwrapped piece of meat on your kitchen counter for several days. Before long, you’d begin to see spots of sundry hues and textures, which, if viewed through a microscope, would resolve into colonies of various microorganisms. They’re all around us—on surfaces, in the air—all the time, and for them that piece of meat is the promised land.
We are meat. The human body is a highly desirable environment for all manner of microorganisms—viruses, bacteria, fungi—to complete their life cycles, replicating and multiplying, and sometimes making us sick in the process. It’s the job of the immune system to find and expel these interlopers before they can harm us.
The first line of defense against such incursions is specialized white blood cells called lymphocytes. They come in two main varieties: B-cells and T-cells. The former comprise the reconnaissance unit. Individual B-cells are tuned to respond to specific surface proteins called antigens, which are characteristic of a particular pathogen. They patrol the bloodstream, and when they recognize a trespasser, they churn out millions of antibodies that seek out the intruder and paint a target on it.
B-cells can’t penetrate cell walls, however, so they’re ineffectual against enemies such as viruses that lurk inside the body’s cells. That’s where T-cells come in. T-cells start out naive, meaning they aren’t programmed to go after a particular quarry. Instead, they get their marching orders from a third type of white blood cell, called dendritic cells.
Edgar Engleman has made a career of elucidating the role of these relatively rare but pivotal players. Named for their treelike branching appendages, “[dendritic cells] are unique in their ability to sense when evil lurks and sound the alarm,” he explains. “Basically they present pieces of those agents, whether they be viruses or bacteria—or tumors—to the other parts of the immune system and essentially say, ‘Go get ’em.’”
Obviously, cancer is capable of evading the body’s immune defenses; otherwise, people wouldn’t get it. But consider this: 9.2 million people donate blood in the United States each year. Out of an abundance of caution, individuals who have had cancer are ineligible to donate for a period of time following treatment. Even so, the average incidence of cancer indicates that upwards of 42,000 donors, unbeknownst to them, have cancer cells circulating in their blood. And yet the instances of someone developing cancer as a result of a blood transfusion are so rare that when they’ve occurred they’ve warranted publication in medical journals as a curiosity.
Cancer may be cunning, but it isn’t catching.
That’s not a trivial observation, says Engleman, who has directed the Stanford Blood Center since its inception in 1978. It’s profound. Think about it: Why would a person’s immune system attack cancer cells from someone else just as vigorously as it would a skin graft or heart transplant, but be blind to a tumor that developed from that person’s own cells?
See, our own cells all carry a sort of molecular banner proclaiming them as “self”—and rule number one of the immune system, ingrained before birth, is don’t attack self. Any cell that doesn’t carry this sigil, whether it’s transplanted tissue or an invading pathogen, is targeted for elimination. That’s why patients receiving an organ transplant are given drugs that suppress their immune response.4
Engleman (Photo: Steve Fisch)
Under normal circumstances, a healthy immune system tends to be conservative. That’s a good thing: A hypervigilant immune system that attacks the body’s own cells as trespassers leads to autoimmune disease. But that also means that, for the most part, tumor cells look enough like the normal self cells that they started out as to fly beneath the immune system’s radar.
What’s more, malignancies are adept at altering the environment around them to make it more hospitable—including hypnotizing the immune system into helping rather than hunting them. “It’s almost impossible for the immune system to make that distinction without a little kick in the butt,” says Engleman.
“That’s what it turned out to take.”
As recently as 25 years ago, the idea of marshaling the body’s own immune defenses to fight cancer was laughable, Engleman says. “We thought, and immunologists taught, that it wasn’t just that our immune systems are reluctant to react to cancer; they can’t, because all of the B-cells and T-cells that are capable of recognizing self have been physically removed and destroyed.”
Yet when scientists looked inside tumors, it turned out they were teeming with immune cells. Only rather than attacking, they had been subverted and the cancer was using them to promote its own growth.
Engleman was among the first to demonstrate that that suppressed state was reversible. In the early 1990s, he had the idea, “which at the time was pretty harebrained,” he says, of removing dendritic cells from a patient with cancer, manipulating them in the lab to arm them with tumor antigens, and then injecting them back into the patient in the hope that they would activate the T-cells to attack the malignant tissue.
The approach differed from the lab-created antibody therapies—such as rituximab for lymphoma (developed at Stanford by Ronald Levy, MD ’68, now chief of the division of oncology at the School of Medicine) and herceptin for breast cancer—that were just becoming available at the time, in that the patient’s own cells would be used to provoke an anticancer immune response inside his or her body.
Engleman and a colleague, Samuel Strober, tried it, initially in lymphoma patients and later in colorectal, lung and a handful of prostate cancer patients at Stanford, using dendritic cells isolated and prepared at the blood center. The results were promising: The armed dendritic cells spurred the patients’ T-cells to go after their tumors. And they did it without causing any incidental problems such as an autoimmune reaction. “It didn’t cure anybody,” Engleman says, “but it worked and it was safe and it could be used in situations where we didn’t have monoclonal antibodies.”
The university’s Office of Technology Licensing filed patents and tried to find existing companies that would license the technology. (See sidebars below.) “Initially none of the pharmaceutical companies were interested in this,” Engleman recalls. “Cell therapy? What’s that about? I think the idea of individualized therapy that’s not ‘drug in a bottle,’ they didn’t get it.”
But a Harvard professor heard Engleman give a talk about the work and put him in touch with some venture capitalists who he said might be interested in funding such a company. In 1992, Engleman and Strober founded Dendreon to develop the technique into a marketable product. The “vaccine” sipuleucel-T—or Provenge, as it came to be known—was the original dendritic cell-based immunotherapy and one of the first concrete examples of personalized medicine.
Because it was unlike anything anyone had ever seen before, the FDA required the company to perform clinical trial after clinical trial, and the treatment languished in regulatory limbo for more than a decade. Meanwhile, Dendreon had sunk enormous investments into manufacturing facilities and infrastructure, which they were apparently unable to recoup when revenues failed to meet expectations. (The cost was on the order of $90,000 per course—no greater than the standard biological therapy, Engleman notes.) The company filed for bankruptcy in 2014; a Canadian pharmaceutical company acquired Provenge and other assets for $415 million early last year.
Though his involvement with Dendreon was limited to an advisory role—and ended 15 years ago when the company relocated to Seattle—Engleman says watching from the sidelines as it unraveled was terribly painful. “I got it started and it was an amazing thing, so I was very proud of it. On the other hand, after that . . . ugh!”
But he has no regrets. “Basically I had a choice: I could either go work for the company or not. I chose to stay at Stanford and continue doing discovery work, and I’m glad I did.”
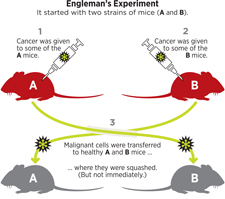
Twenty years later, Engleman still believes that dendritic cells hold the key to rallying the immune system to fight cancer. In 2011, he set up a four-year experiment to model the scenario of accidental tumor transplant (via blood transfusion) in the lab. He took two strains of mice, call them A and B, and gave some individuals of each strain cancer. Then he transferred malignant cells from strain A to healthy strain B mice and malignant cells from strain B to healthy strain A mice.
As predicted, the transferred tumors were quashed by the immune systems of the recipient mice. But it didn’t happen immediately; it took about three weeks.
When Engleman looked more closely at what was going on, he discovered that the recipient mice of both strains had antibodies circulating in their systems that were ready-made to recognize and bind to the foreign tumor. That was a surprise, he says. “I can’t explain to you why Mother Nature decided to give us antibodies to these tumors, but trust me, they’re there.”
Next, he tried extracting these antitumor antibodies from strain A mice and injecting them into strain B mice—and vice versa—with the aim that the donor antibodies would bind and initiate removal of the recipient’s tumors. It didn’t work, but the negative result proved to be illuminating.
It turns out, Engleman explains, that the antibodies themselves don’t kill the cancer. Rather, they initiate a multistep process, mediated by dendritic cells, that ends with specialized T-cells destroying the tumor. “What [the antibodies] did was bridge [the malignant cells] to dendritic cells . . . and sort of go, ‘Hey, dendritic cells, you should eat me.’”
But when cancer is present, the dendritic cells act as if they were asleep at the wheel, he says: They don’t respond to the antibody signal to engulf and metabolize the tumor, they don’t present the tumor antigens to the T-cells, and so the T-cells don’t mobilize.
So to counter the soporific effect of the cancer, Engleman gave the dendritic cells a “wake-up” jolt, in the form of substances known to stimulate them, as an adjunct to the antibodies. The result was astounding. “When we gave the two things at the same time, BOOM, then we got an explosive antitumor immune response that cured the tumors in the mice. Reproducibly, over and over again.”
Not only did the combination injection kill the primary tumor, but it wiped out distant metastases as well. That’s huge because, as any oncologist will tell you, in most cancers it’s the metastases that are deadly. Moreover, there was no autoimmune reaction, no toxicity, no collateral damage whatsoever. The results were published last spring in the prestigious journal Nature.
Because the antibodies are present in all healthy individuals (albeit in varying concentrations) and the stimulant substances are commercially available, in theory the approach should be eminently scalable, Engleman says. It should also be far less expensive than individually tailored treatments like sipuleucel-T or the more recent chimeric antigen receptor T-cells (CAR T), an approach in which a patient’s T-cells are isolated and genetically manipulated to give them a new receptor that targets an antigen on cancer cells.
Of course, there’s no guarantee that the treatment will be as safe, effective and well tolerated in humans as it was in the mice, he cautions. “I have to point out that the mice rarely protested; they didn’t fill out complaint forms and tell us how miserable they were.”
“Many of our therapies today are very good at removing the ‘brake’ from the immune system . . . and making our cells do their job better,” says Holbrook Kohrt, MD ’04, PhD ’12, an assistant professor in the division of oncology at the School of Medicine and translational clinical trialist specializing in cancer immunotherapies. Other therapies, by the same analogy, press down on the gas, boosting the activity of “killer” T-cells.
“What we have not been able to do,” he says, “is to effectively help our immune system target our tumor cells.” That is, steer. “Ed’s technology does that.”
It also demonstrates “that you don’t necessarily have to be perfectly personalized in order to induce a perfectly personalized immune response,” Kohrt says. By modulating dendritic cell function inside the body to a large degree, the technique reduces the reliance on complicated manufacturing processes, “sav[ing] incredible amounts of time, of money and of infrastructure.”
Citing five- and 10-year survival curves for melanoma as an example, Kohrt notes that the first generation of cancer immunotherapies raised the survival rate to 16 percent (versus 8 percent with standard chemotherapy). With the second generation, the rate went up to about 24 percent, and with the current generation, he says, it might be possible to get overall survival up to 50 percent. “But there’s still a lot we’re leaving on the table. And one area where we’re not going, an area that has not been successful—yet—is the area that Ed’s technology directly goes into.
“I think it’s pretty obvious that this is going to be big as long as it’s done so the science leads it. And Ed has always been somebody for whom the science has led where he goes in the lab.”
Before he died last fall, Engleman’s father, Ephraim, ’33, a rheumatologist who maintained his practice at UCSF well past his 100th birthday, asked him, “Why aren’t you already treating patients; what’s the matter with you? I don’t understand, aren’t you interested?” To which the younger Engleman replied, “Dad, it’s not that easy; it costs a lot of money.”
The path from a petri dish to a physician’s prescription pad is an interesting and daunting challenge, agrees Mike Alonso, who has wiggled his toes in the grass on both sides of the academia/industry fence. As a doctoral student, Alonso, PhD ’11, worked with a collaborator who was spinning out her technology. He joined the startup, called Organ-i, which was developing a molecular diagnostic test to determine whether an organ transplant recipient would develop acute rejection.
But after a year or so, the lab beckoned. “As awesome as it is, it’s tough to be singularly focused on one thing,” he says of his time with the company. “Especially once you realize that thing can be improved dramatically.”
Now a research associate in Engleman’s group, Alonso is leading the charge to move the “alloantibody therapy with dendritic cell stimulus,” as it’s technically called (allo- basically just means “someone else’s”), into human clinical trials as expediently as possible.
Typically the first hurdle is translating the technology between species, e.g., from a mouse to a human, he says. “There are a number of variables involved there: Most notably, will the technology behave as you expect it to? In this case, we got lucky because a lot of those mechanisms are fairly well conserved.”
As of late last year, Alonso was working with human cell lines in vitro and peripheral blood from healthy volunteers at the blood center to modify the antibody preparation and screen a variety of commercially available stimulants that are FDA approved or in late-stage clinical trials to try to find the optimal combination. The hope being that if the components are already approved, the FDA won’t make the Engleman lab do toxicology studies. But the agency may require such studies to be completed anyway. Or the ideal components may not be commercially available, in which case they may take millions of dollars to develop.
Researchers also need to work out the delivery method, says Engleman. In mice they injected the antibody-and-adjunct preparation directly into the primary tumor, but not all tumors are so easily accessible. From there, whether the Phase I human clinical, or safety, trial will take place at Stanford is an open question, he says. But beyond that, “the resources, the expertise [to do drug development], generally don’t exist” in a university environment. At some point, “we need to get industry involved to be able to carry it all the way through.”
It’s a road he’s been down before. Still, “having lived through other approaches, this looks like a really good one, and I’m hoping that it can be translated into humans in my lifetime,” Engleman says. “Make my father proud.”
Where Inventions Go Next
Established in 1970, the Office of Technology Licensing (OTL) plays an important role in managing how knowledge created on the Farm is shared with the wider world. Its mission is to help turn scientific progress into tangible products, while generating income back to the inventor and university to support more research. The office evaluates hundreds of invention disclosures each year to determine whether to pursue intellectual property protection (copyrights, patents). It also works with the creator to identify entities that might pay for the right to make use of a discovery—whether it’s a researcher at another university who wants a fluorescent molecular tag or a startup that wants the code for a data visualization algorithm. The OTL receives 15 percent of the money from these agreements for its own operating costs. Of the remainder, a third goes to the inventor, a third to his or her department, and a third to his or her school. In the 2013-14 fiscal year, this amounted to $22.1 million directly to Stanford researchers, $20.5 million to individual departments, and $18.4 million to the various schools. Departmental and school shares are unrestricted funds that can have a big impact on innovation, particularly as the federal government has shrunk grants for research.
By the Numbers
Among leading U.S. research universities, Stanford ranks:
5th in number of patents filed annually in 2013 (298)
7th in number of licenses and options issued annually (123)
8th in gross annual licensing income ($86,967,392)
Most inventions are never licensed.
The OTL receives 9 or 10 invention disclosures per week:
50% have patent applications filed
20% to 25% are licensed*
In 2013-14, approximately:
47% of disclosures were in the life sciences
34% were in the physical sciences
11% were in the joint physical and life sciences
8% were medical devices
Stanford’s top three all-time earners:
Process to create functional antibodies (1984): $486 million
Improved hypertext search algorithm, aka Google (1996): $340 million
Recombinant DNA (1974): $255 million
* Certain inventions, such as software and biological materials, are licensed without patent protection. Based on FY 2013 data. Sources: Association of University Technology Managers (AUTM) Statistics Analysis for Tech Transfer (STATT) database; Stanford OTL
Greta Lorge, ’97, is a Bay Area writer and editor.